AG Photo luxury
Introduction
In photography, knowing light is essential and, in this chapter, I will try to explain as simply as possible this complex phenomenon. Describing light has always been an arduous task, and before the advent of quantum mechanics, it had not always been adequately described. The first description of light or more generally of electromagnetic radiation (which light is a part of), depicts it as a particle that moves from point A to point B. Scientists had imagined then that light might be composed of small moving particles. Subsequently, it was noted that describing light as a particle did not explain certain behaviors, and this was insufficient. One of the behaviors that was not explained was diffraction. Because of this, light was described as a wave in order to resolve this problem, but it brought about another: the propagation of light in a vacuum.
In fact, any wave phenomenon is unable to propagate itself in a vacuum. A wave is an energy transmission from point A to point B and not of matter.
In practice, energy that is transmitted between them through vibration moves, not the particle. Therefore, if between points A and B there are particles 1 through 4, then when particle 1 vibrates, it makes particle 2 vibrates. This in turn causes particle 3 to vibrates, which then causes particle 4 to do so as well. The consequence is that a wave of energy is transmitted from point A to point B, but none of the particles has changed his position.
Therefore, in the vacuum of space (where we can assume that there are no particles), the propagation of light should be impossible if it were a wave.
For many years there have been experiments to see if the light was one or the other thing, but some of these were showing light as a wave while the others were showing it as a particle.
None of the experiments were ever able to demonstrate that it was, in reality, a phenomenon that had both characteristics at the same time.
In the end, quantum mechanics was able to definitively explain light as phenomenon with wave and particle characteristics.
Today, light is described as a discrete phenomenon (not continuous) that can assume only some values of energy, multiples of those associated with its fundamental particle: the Photon.
A photon has an electric and magnetic field that is perpendicular to them (figure 01).
01 In this figure we see the electromagnetic field and the electricity generated by the moving photons.
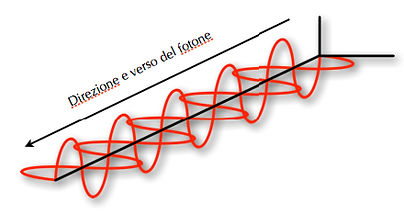
After Einstein published “The Photoelectric Effect”, which earned him a Nobel Prize, light was no longer describe in this way. Now it was observed that beam electrons behaved like waves and particles and had an electromagnetic field associated with it. In figure 02, we can see a wave (in red) and its main features.
02 In this figure we can see the three fundamental characteristics of a wave: its length (distance between the two crests), amplitude and frequency.
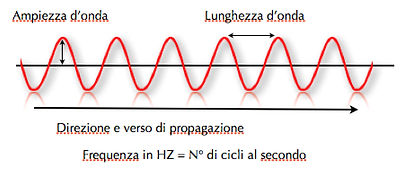
The wavelength is linked to the frequency by this relation, λ=c/ν, where λ is the wavelength in meters (m), “c” is the speed of light in millimeters/second (m/s) and “v” is the frequency in hertz (Hz). We use the speed of light, c, only for electromagnetic waves.
In order to explain better, we can see in figure 03 where it shows how, going from left to right, by increasing the energy of the electromagnetic wave that the it increases the frequency while decreases the wavelength, λ. The electromagnetic radiation always travels at the speed of light, but, when the wavelength diminishes, it completes more cycles in the same space. In order to maintain its speed, it has to increase its energy.
Introduction
The camera
How it is made a Reflex camera
Photographic lenses
Equipment and accessories
Take a picture:
Buyer’s guide
03 λ=c/v The electromagnetic wave, in red, from left to right, increases its energy and frequency by decreasing the wavelength.
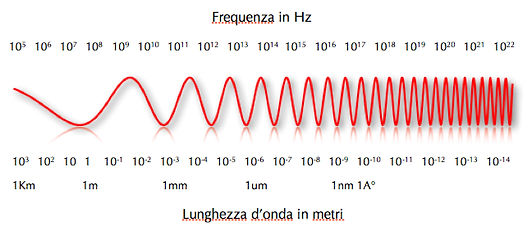
In figure 03 (at the bottom of the figure), we see how the wavelength passes in size from kilometers (km) to thousandths of nanometers (nm). The wavelength, λ, also determines the penetrability of the wave in the material. In figures 04 and 05, we can understand that by decreasing the λ we can increase the penetrability. The more penetrating the wavelength is, the more damaging it is to one’s health. Electromagnetic waves become dangerous for our health when λ is equal to or less than 10-7, or rather by UV rays (λ<10-7m), moving towards the right of the spectrum.
04 λ large, low energy and penetrability in the material.
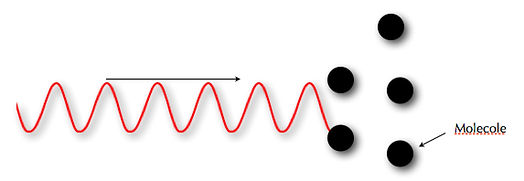
05 λ small, high energy and penetrability in the material.
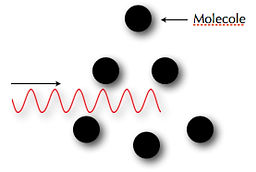
Electromagnetic radiation spectrum
Often, when we talk about light, or more generally about electromagnetic radiation, we also hear the word “spectrum”. The electromagnetic radiation spectrum indicates all of the wavelengths together that can be expressed. In figure 06, we can see the complete spectrum. The figure should be read from left to right exactly like in figure 03.
Little by little as we move to the right of the figure, the energy increases, thus increasing the frequency and decreasing the wavelength. We can see, at the bottom of the figure, how different wavelengths correspond to different technological uses of the wave. Waves used to transmit radio, television, radar and infrared are not dangerous to man because they are not penetrating. Neither are microwaves dangerous at low power. They become dangerous, in the case of microwave ovens and with cellphones, because they are highly concentrated.
Infrared radiation is emitted by warm bodies and it is therefore useful to see human beings in the dark by using the appropriate device (our eyes cannot see infrared radiation). To the right of the value of λ at 10-7m, we enter into the danger area for humans with UV rays emitted by the sun (but stopped by ozone), X-rays used at times at the doctor’s office and lastly, gamma rays emitted by celestial bodies, by radioactive material or following the explosion of a nuclear fission device.
Of all of these wavelengths, our eyes can see only a small portion: this portion is called “the spectrum of visible light”. This spectrum is when the wavelength (λ) is between 10-6 and 10-7m. In figure 06, the zone has been enlarged otherwise it would have been extremely insignificant when shown with all the colors.
The difference between one color with respect to another is simply the wavelength.
Therefore, in photography, the characteristics of light that interest us the most is the wavelength, because it is the one that determines the color. Light that is called “white”, that we see coming from the sun in the atmosphere, is a combination of photons of different wavelengths that are moving across the spectrum of visible light: from red, at the lowest energy and wavelength, to violet, which has the highest frequency.
06 The complete spectrum of electromagnetic radiation.

Seeing colors
Human beings have eyes that are sensitive to light wavelengths between 10-6 and 10-7m. Inside this spectrum, our eyes distinguish single colors with great sensitivity.
We are able to see the world around us, because the light hits object that surround us that then arrives at our eyes.
Imagine being in the room of an apartment during the day. The light of the sun enters the window and hits, directly or indirectly, all the objects and the people inside. The white light of the sun that enters from the window is comprised of all of the wavelengths of the visible spectrum (and also others that we cannot see).
When the white light hits a table, the wood absorbs different wavelengths and transmits instead that light which corresponds to brown. By having absorbed the others, the only thing that arrives at our eyes is the “brown” wavelength. Therefore, we only see the table as this color.
But not all light is similar to the sun light. If we take, for example, light that comes from an incandescent bulb with a tungsten filament, it is comprised of different wavelengths but not the same wavelengths that come from the sun. In lamplight, some wavelengths are missing in the blue and violet zones, and, therefore, the table transmits a different color than what we might see in the presence of sunlight. Some frequencies cannot transmit them, because they are not present in the light source.
With some types of illumination, we perceive only a variation in the color gradation while with some particular light sources, the colors of objects can change a lot depending on the components of the light.
Every material has the ability to absorb a specific spectrum that includes also the wavelength outside of the visible spectrum. Because of this, the resulting color of an object is due to all of the wavelengths minus the spectrum of light absorbed by the object. The color that we see is due to only those wavelengths that fall between the λ of 10-6 and 10-7m.
But besides this, we have to also consider that our perception of color can be altered by our own brain (see the chapter on white balance in the paragraph entitled “Colors and our perception of them").